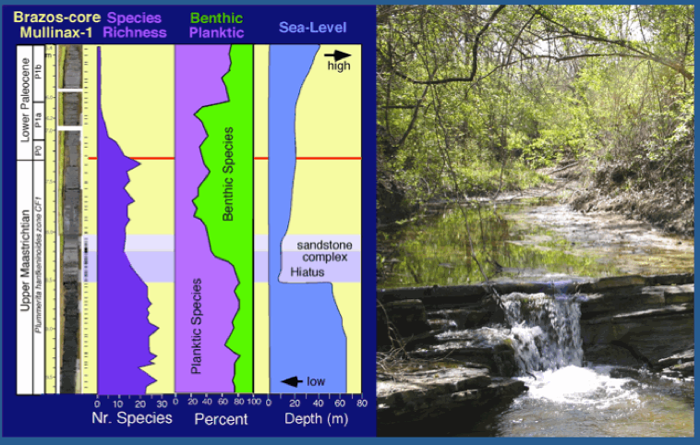
Figure 69. Illustration of sea level fall and rise near the end of the Maastrichtian led to depositing of the sandstone complex in the Brazos sections as seen in the Darting Minnow Creek tributary. Benthic and planktic foraminifera show the dramatic change associated with this sea level fall in the shallow environment of the Western Interior Seaway. From Keller 2007.
Iridium
After 30 years of chasing evidence in support of the Chicxulub impact as the cause for the KTB mass extinction we found little more than the iridium anomalies, which are usually very small (<0.5 ppb), except for rare locations in Italy (Gubbio), Denmark, Tunisia (El Kef), India (Meghalaya). They are never associated with undisputed Chicxulub impact ejecta (impact spherules), and frequently occur in multiple small Ir concentrations at clay layers above and/or below the KTB. Iridium concentrations such as these are increasingly being questioned as primary sources and re-interpreted as remobilization and re-concentration at redox layers (Colodner et al., 1992; Martin-Peinado and Rodriguez-Tovar, 2010; Miller et al., 2010; Gertsch et al., 2011). The Ir contribution from volcanism, formerly thought to be negligent, is also under study.
Impact-tsunami
The impact-tsunami hypothesis fared worse. Invented to explain the wide separation between impact spherules at the base of a sandstone complex and the KTB mass extinction above it, this hypothesis quickly became the panacea to explain every non-conforming sequence as impact-generated tsunami. In this exuberant enthusiasm even the KTB was redefined as the level of any Chicxulub impact ejecta leading to perfect circular reasoning. Chicxulub had become KTB age by definition. But defining the Chicxulub event as KTB age doesn’t make it so. The evidence, the ground truth, contradicts this claim. There are no confirmed impact-tsunami deposits. Indeed, research around the world on recent tsunamis has revealed that deposits generated by tsunami waves tend to be redistributed within hours, days and weeks with no evidence remaining in the long-term.
The sandstone complex below the KTB in NE Mexico, Chicxulub crater and Texas that is commonly interpreted as impact-tsunami generated contains numerous evidences that show long-term deposition, including multiple impact spherule horizons separated by sand or limestone with burrows (section 4), lithified clasts with impact spherules that indicate deposition at an earlier time followed by erosion, transport and re-deposition (section 10), invertebrate burrowing horizons that indicate colonization of the ocean floor during depsosition (sections 4, 10), multiple zeolite (ash) horizons that indicate discrete deposition in quiet waters over time (section 4), multiple horizons of glauconite (Chicxulub crater core, section 5) indicating deposition over tens of thousands of years on a quiet seafloor. All of these features are characteristic of long-term deposition and incompatible with the tsunami hypothesis. “How could so many be so wrong for so long” is the frequently asked question. The answer is an exuberant belief in the impact hypothesis as the only explanation for the KTB mass extinction led many scientists to throw out the baby with the bathwater. The evidence was always there, but disregarded, thrown out or re-interpreted as impact-generated.
Sea-level change
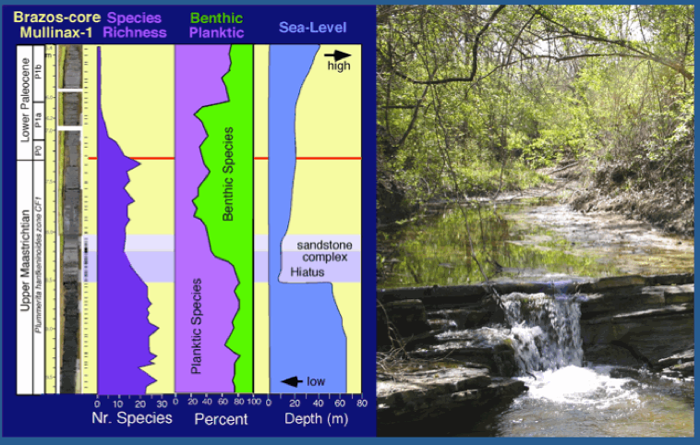
Figure 69. Illustration of sea level fall and rise near the end of the Maastrichtian led to depositing of the sandstone complex in the Brazos sections as seen in the Darting Minnow Creek tributary. Benthic and planktic foraminifera show the dramatic change associated with this sea level fall in the shallow environment of the Western Interior Seaway. From Keller 2007.
All the evidence of the sandstone complex can easily be explained by sea-level change, the rise and fall that result in a transgression and regression, respectively. During a low sea level submarine channels are soured, subsequently sediments are eroded and transported from shallow areas into the deep via submarine channels. Normal marine sediment deposition resumes during the sea level transgression. This is observed in the sandstone complex of NE Mexico and Texas and elsewhere. Sea level changes such as these occur through history. The only reason the latest Maastrichtian sea level fall has garnered so much attention is because this deposit has impact spherules (eroded and transported) and it is close to the KTB.
In NE Mexico, the sandstone complex is in deep water on the continental slope (500-1000m depth, see Fig. 19). In Texas, the sandstone complex was deposited in a shallow marine environment. Nonetheless, the depositional sequences are similar and both mark the sea level fall that accompanied the global cooling near the end of the Maastrichtian after the greenhouse warming in C29r, planktic foraminiferal zones CF1-CF2. An estimate of the sea-level change can be seen in Fig. 69 based on benthic foraminifera.
Planktic and benthic foraminifera are good proxies for assessing sea-level changes particularly in shallow waters (Fig. 69). Planktic species are most diverse and abundant in open marine environment where they occupy diverse niches from the surface to deep. When sea level drops the deepest dwellers in relatively shallow environments loose their habitats and consequently species diversity and population abundances drop. In contrast, benthic foraminifera increase their diversity and abundance in shallow areas. Therefore, the abundance of benthic and planktic species over time is a good indicator for sea-level change. At the Brazos sections the effect of the sea level drop coincident with deposition of the sandstone complex is seen in the dramatic decrease (45%) in the number of planktic species and a major reduction (from 80% to 20%) in the species populations (Fig. 69). At the same time benthic species increase in abundance from 20% to 80%. Above the sandstone complex, sea level begins to slowly rise through the latest Maastrichtian and the KTB into the early Paleocene.
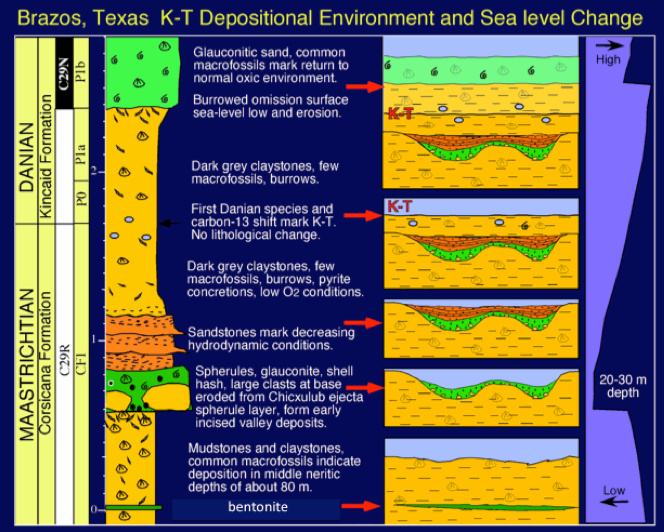
Figure 70. Illustration of scouring of seafloor during a sea level fall and the infilling of the submarine channel subsequently as the sea level rises again. From Keller 2007.
A simple illustration shows the effects of a sea level fall, the scouring of the seafloor creating submarine channels, and the subsequent infilling when sea level rises again. The sequence begins at the bottom of the graph with normal deposition, including a bentonite/ash layer. At the maximum sea level fall (regression) the seafloor is scoured creating channels through which sediments eroded in near shore areas are transported seaward into deeper waters. Frequently larger clasts ripped up from the seafloor line the channel bottom. When sea level begins to rise the first sediments eroded from the shore are deposited in these submarine channels. In Texas and Mexico these sediments are generally shallow water debris, wood, plants, and shallow water benthic foraminifera, glauconite and abundant impact spherules (green) (Fig. 70). Several of these layers may be deposited at different times separated by sandstone or limestone as in Texas and NE Mexico.
As the sea level rise continues and transgresses landward the distance to the depositional center increases. Finer sand is transported and deposited either as more massive sands via gravity slumps in deep water, or as hummocky cross-bedded sandstone (HCS) in shallow waters, such as in Texas. Some time passes before the next layers are deposited because the HCS is burrowed and burrows are truncated by erosion. With the rising sea level finer sands are alternating with shale and clay layers are deposited. These are invariably strongly burrowed marking colonization of the seafloor during deposition. With still higher sea level normal sedimentation resumes as the depositional center is out of reach of all but normal fine sediment transport. In the Texas sections this level is reached well before the KTB.
This scenario is illustrated for two Brazos sections along with stable isotope data that marks the global cooling coincident with deposition of the sandstone complex (Fig. 71). Above the sandstone complex, Maastrichtian isotope signals and planktic foraminiferal assemblages in claystones indicate that sedimentation resumed during the latest Maastrichtian. The KTB is marked by the characteristic drop in carbon isotopes, the mass extinction and evolution of first Danian species (Fig. 71).
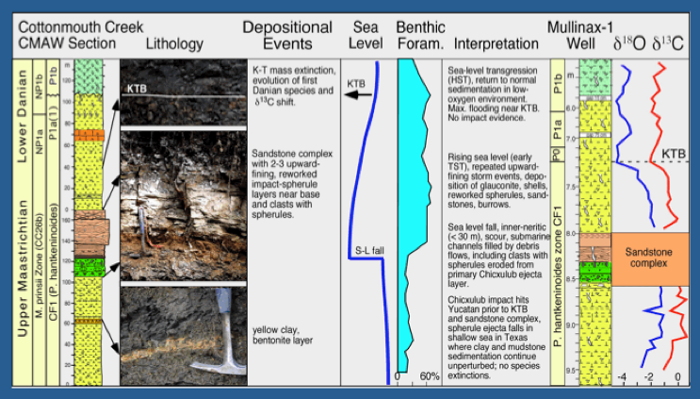
Figure 71. Deposition of the sandstone complex, carbon and oxygen isotopes and sea level changes in two sequences along the Brazos River and its tributary the Cottonmouth Creek. From Keller et al., 2011.
The ultimate proof
The problems with the impact-tsunami interpretation for the sandstone complex in Mexico and Texas and the Chicxulub crater core were not lost on all. The ultimate proof that the Chicxulub impact was KTB age and caused the mass extinction would ideally come from marine sections where the impact spherule layer is precisely at the KTB and there is no complication with channelized deposits.
This proof was believed to have been found on Blake Nose (ODP Sites 1049A-C) Carribbean (ODP Sites 999, 1001) and Demerara Rise (ODP Site 1259) (section 9).
Early studies of these sections concentrated on the juxtaposition of Paleocene and Maastrichtian sediments with a 2-15 cm thin spherule layer in between, but failed to recognize the major unconformity present (see section 9). In all but the Demerara site is the late Maastrichtian interval of zone CF1-CF2 missing and in the Caribbean sites most of the late Maastrichtian is missing. In addition, all these localities miss most of the early Danian P. eugubina zone. The spherule layer contains abundant early Danian species along with reworked Cretaceous species. Deposition of the spherules thus occurred in the early Danian a long time after the KTB mass extinction. The impact spherules are thus eroded from an earlier older deposit, transported and redeposited in the Danian (Keller et al., 2013).
Geographic distribution of impact spherules
What can the geographic distribution of impact spherules tell us? If the distribution is random regionally as well as stratigraphically, then not much can be said. But if there is a pattern to it, this can tell a lot. Our team has examined well over a hundred sections in more than 65 localities over the past 20 years. The spherule distribution is remarkable. All localities in the N. Atlantic and Caribbean, the Islands of Cuba and Haiti, the countries of Belize, Guatemala and southern Mexico have impact spherules only in the early Danian at least 100 ky after the KT boundary (Fig. 72). In Central and Northeastern Mexico aspherule layers are always found only in the latest Maastrichtian zone CF1, which spans the last 160 ky below the KTB.
This remarkable distribution pattern reflects Gulf Stream erosion. All localities with impact spherules reworked in the early Danian (zone P1a) sediments are positioned in the path of the Gulf Stream current. In these localities erosion and non-deposition by far exceeds sedimentation through the Cretaceous and Paleogene (Keller et al., 1993). Given this fragmented record due to Gulf Stream erosion, plus Caribbean tectonic activity, there is little hope of finding a complete record with the primary Chicxulub impact spherule deposits in the North Atlantic. It is far more likely that impact spherule deposits were reworked and redeposited into younger sediments by intensified currents activity and tectonically induced earthquakes as high-resolution stratigraphy indicates (Fig. 72).
However, the remarkably consistent stratigraphic age distribution of frequently multiple impact spherule layers in zone CF1 below the KTB in submarine channels of Central and NE Mexico and Texas is neither random nor impact controlled (sections 4, 10). The common factor is the latest Maastrichtian sea level fall, which occurred in the upper part of zone CF1 and resulted in the widespread down-cutting of submarine channels that were subsequently infilled by a sandstone complex with reworked impact spherules at the base (frequently interpreted as impact-tsunami). These spherule layers are reworked from older primary spherule deposits in nearshore areas (as indicated by the presence of shallow water debris, plants, shallow water benthic foraminifera) and transported seaward. In many sections the presence of multiple reworked spherule deposits separated by marls or limestone with burrows indicate reworking occurred over an extended time period. The overlying sandstones, alternating fine sand, silt and marl layers that infill the submarine channels contain abundant burrows and trace fossils that also indicate deposition over an extended time period that is not compatible with the popular Chicxulub impact-generated tsunami hypothesis.
Given the multiple factors (currents, erosion, slumps, tectonic activity, earthquakes) that affected sedimentation through the North Atlantic and Caribbean finding multiple layers of reworked impact spherule deposits is common, but finding primary impact spherule deposits is difficult and rare. We discovered an apparently primary impact spherule deposit was discovered at El Penon, NE Mexico, in a 2 m thick spherule layer over 4 m below the reworked multiple spherule layers at the base of the sandstone complex (sections 6, 8). This spherule layer differs from all others in the absence of shallow water debris, presence of calcite cement between spherules rather than other debris, deposition while glass still hot as indicated by convex/concave contacts and strongly compressed and flattened spherules. The age of deposition is near the base of zone CF1, or about 100 ky before the KTB (Keller et al., 2009).
At El Penon the planktic foraminiferal record shows no mass extinction or any other significant effects as a result of this impact (sections 6, 8). This indicates that the environmental effects of the Chicxulub impact were short-term and cannot be detected at the 500-1000 year scale of our sample resolution. It also means the catastrophic effects of the Chicxulub impact have been vastly overrated and that this impact could not have caused the KTB mass extinction. Another catastrophe must have caused the KTB mass extinction. Increasing evidence points to Deccan volcanism as this other catastrophe.
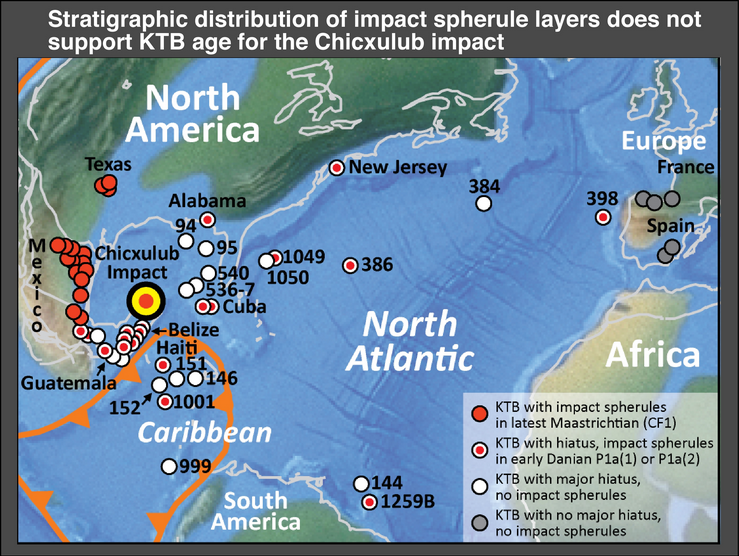
Figure 72. Paleogeography of the North Atlantic and Caribbean during the Cretaceous-Tertiary transition with paleolocations of all KTB sections with impact spherules. Note the consistent stratigraphic distribution of impact spherule layers in the latest Maastrichtian zone CF1 about 100 ky below the KTB in NE Mexico and Texas. In all other localities, such as the North Atlantic, Caribbean, Cuba, Haiti, Belize, Guatemala and SE Mexico, impact spherules are reworked in early Danian sediments P1a(1) or P1a(2) about 100 ky after the KTB overlying hiatuses of variable extent. This distribution pattern is likely due to intensified Gulf Stream erosion, which did not reach NE Mexico and Texas. Modified after Scotese (2000) andKeller et al. (2013).