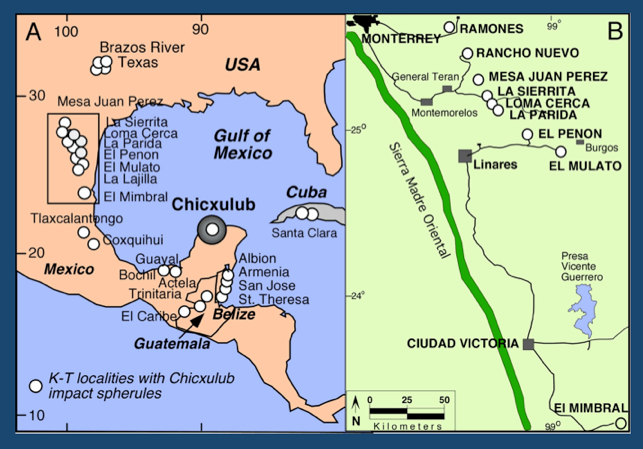
Figure 10. A. Localities with impact spherules surrounding the Chicxulub impact crater. B. Impact spherules in upper Maastrichtian sediments in NE Mexico. Deposition occurred in submarine channels. From Keller et al., 2009.
The inconclusive age control of the Chicxulub crater in the early 1990s placed the burden of proof on sections with impact ejecta (e.g., iridium, impact glass spherules) surrounding the Gulf of Mexico. But here too, problems were apparent from the very beginning. The first discovery of impact glass spherules in northeastern Mexico came from El Mimbral and El Peñon and subsequently from over 65 other localities (Fig. 10).
In nearly all localities a thick sandstone complex infills submarine channels along the slope of the western Gulf of Mexico where eroded sediments from the rising Sierra Madre Oriental are carried into the Gulf and transported downslope via submarine channels. The channels are generally about 100-300 m wide and can be traced laterally in outcrops. Sediment deposition is similar in most channels and commonly divided into three distinct: Unit 1 at the base consists of a glauconite and impact-spherule rich debris enriched with shallow water benthic foraminifera, wood and plants. The middle Unit 2 consists of thick sandstone. The top unit 3 consists of alternating layers of sand and marly shale (Figs. 11, 12, 13). All three units together constitute a sandstone complex, which is commonly labeled “impact-tsunami” deposit by proponents of this hypothesis.
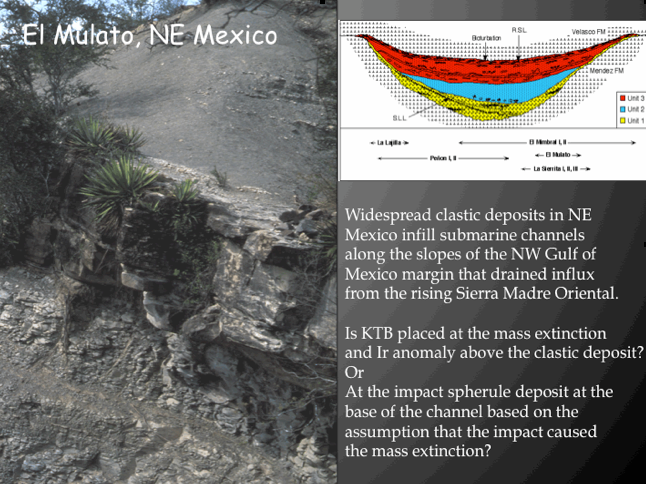
Figure 11. Sandstone complex at El Mulato.
The first discovery of the sandstone complex with impact spherules (unit 1) in Mexico was at El Mimbral (Alvarez et al., 1992; Smit et al., 1992). Subsequently, the best locality was discovered at El Penon. Numerous localities were discovered with multiple impact spherule layers always in the uppermost Maastrichtian and at the base of submarine channels (e.g., El Penon, La Lajilla, El Mulato, Loma Cerca, Mesa Juan, La Sierrita and many others). Most localities show similar lithology and biostratigraphy and all have impact spherules in the latest Maastrichtian below the KTB.
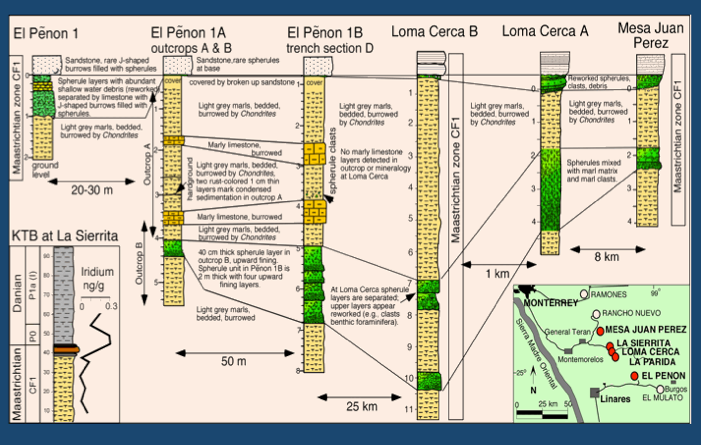
Figure 12. Chicxulub impact spherules in late Maastrichtian submarine channel deposits in NE Mexico. Note these multiple impact spherules layers are generally reworked from shallow nearshore areas, except the deepest deposits, such as at El Penon which show no shallow water debris. From Keller, 2009.
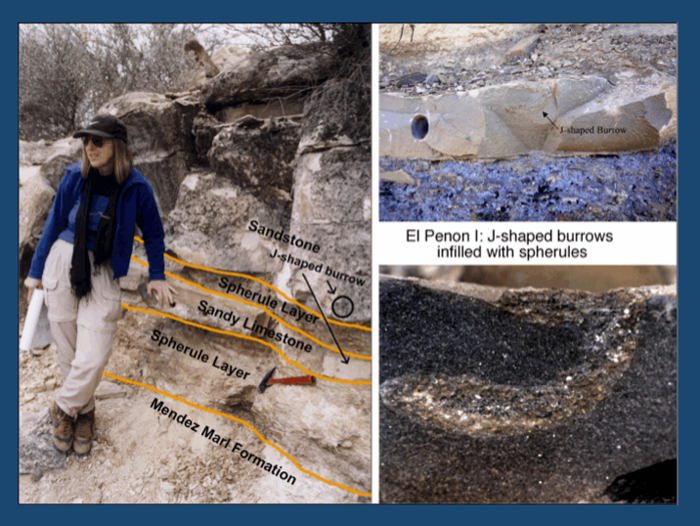
Figure 13. El Penon outcrop showing lower and upper impact spherule layer separated by sandy limestone (close-up seen in upper right) with rare J-shaped burrows infilled with impact spherules (lower right). From Keller et al., 2004.
At El Mimbral and El Penon, a glauconite and impact spherule-rich unit 1 is at the base of a sandstone complex that infills submarine channels at a paleodepth of about 500m. This unit1 is about 1m thick but separated into two layers by a 25 cm thick sandy limestone with an undulating erosional surface at the top and occasional J-shaped burrows infilled with impact spherules (Fig. 13). Above the sandstone complex, a small Ir anomaly was detected at El Mimbral at a redox layer coincident with the mass extinction of planktic foraminifera (Keller et al., 1994b).
If the Chicxulub impact caused the KTB mass extinction and the Ir anomaly, then impact spherules should be in close stratigraphic proximity. Instead, at all localities they are widely separated from 4 m to 12 m. How could this stratigraphic separation be reconciled?
Simple. Assume that the spherules, the Ir anomaly and the mass extinction originated from the same event, then the sandstone complex must be interpreted as deposited at the same time by an impact-generated tsunami (Alvarez et al., 1992; Smit et al., 1992, 1996; Smit, 1999). By this scenario, the spherules rained from the sky within minutes to hours of the impact and settled on the ocean floor. Within hours, impact-generated tsunami waves caused tremendous destruction, margin collapse, and slumps around the Gulf of Mexico, depositing the massive sandstone unit. Within a few days the waning waves of the tsunami deposited alternating silt and marly shale layers. At last the settling of fines and iridium marked the KTB and return to normal conditions. A tsunami interpretation had already been proposed for the sandstone complex and small Ir anomaly that underlies the mass extinction along the Brazos River in Texas (Bourgeois et al., 1988). It was all beautifully simple, and intuitively made sense. But was there evidence for it?

Figure 14. The commonly cited impact-tsunami interpretation of Smit et al., 1992 and Schulte et al., 2010. The evidence contradicts this scenario.
Impact – Tsunami Lacks Supporting Evidence
The impact-tsunami hypothesis could not account for the ground truth. Too many facts contradict tsunami deposition for the sandstone complex in northeastern Mexico and elsewhere (Stinnesbeck et al., 1993; Keller et al., 1997; 2003a,b). Here is a summary of the evidence and the arguments that have been marshaled to either make this evidence consistent with the impact-tsunami hypothesis or simply deny its existence.
Evidence: A 20-25 cm thick limestone layer with an erosional surface at the top and rare J-shaped burrows infilled with impact spherules (Fig. 15B, C). This limestone layer has been observed in spherule rich deposits over a distance of 300 km. |
Interpretation: The limestone layer represent deposition over a long time period, possibly tens of thousands of years. The rare J-shaped burrows infilled with impact spherules (Fig. 15A,B) from the overlying spherule layer indicate living and burrowing invertebrates on the sea-floor where impact spherules are already present and infill the burrows. The sculpted upper surface marks erosion (Fig. 15C). All these features are inconsistent with tsunami deposition over hours to days (Keller et al., 1997, 2002; 2003a). |
Argument in favor of impact-tsunami deposition: Smit and others and Schulte et al. (2010) argued that impact-generated earthquakes of magnitude 11 injected the limestone into the spherule layer (no large-scale disturbance is evident in NE Mexico or elsewhere. Only some small local slumps spanning a few meters have been observed by us or others). Note that no explanation is given for the widespread presence of the limestone layer or the fact that there is no similar limestone elsewhere in the stratigraphic sections from where it could have originated. They further argue that there are too few burrows in this limestone to be of significance, and that the J-shaped spherule-filled burrows are nothing but fluid-escape structures. (Smit, 1999; Smit et al., 1996; Schulte et al., 2006, 2008; Arenillas et al., 2006). |

Figure 15. Limestone layer within the spherule layer at El Penon and El Mimbral. Note the sculpted top of the limestone layer at El Mimbral (C) and the J-shaped burrow at El Penon (A, B). From Keller et al., 2002, e2003.
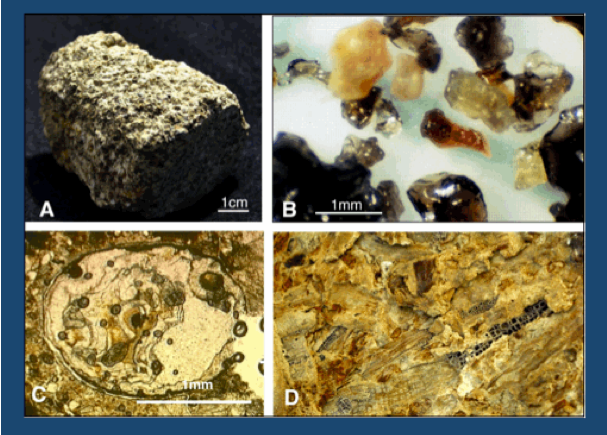
Figure 16. Characteristics of the spherule layers: A. rock of the spherule-rich layer, B. Remnant impact glass preserved. C. Thin section micrograph of spherule in debris matrix. D. Wood and plant debris. From Keller, 2012.
Evidence: shallow water benthic foraminifera (Alegret et al., 2001, 2002), plant and wood debris, clastic sediments and glauconite are present in the spherule layers (Fig. 16). |
Interpretation: this evidence indicates a nearshore source where currents eroded and transported sediments into deeper waters via submarine canyons to a depth of 500 m. This must have happened a considerable time after the impact spherule fallout and probably during a sea level regression. This is not consistent with the tsunami hypothesis. |
Argument in favor of the impact-tsunami hypothesis: Recently, Schulte et al. (2010) argued that the presence of shallow-water benthic foraminifera “contradicts a long-term depositional sequence… because their presence requires unrealistically rapid relative sea-level changes of > 500 m.” Therefore, they argued the sandstone complex could be explained only by impact tsunami deposition. It is rather curiously naïve and absurd for a sedimentologist to argue that the only way to get shallow water debris into deeper waters is by an instantaneous sea-level fall or a tsunami. These impact-tsunami proponents seem to view normal sedimentary processes of erosion, transport and redeposition associated with sea level changes as outside the realm of the possible. |
Evidence: Alternating silt and marly shale layers characterize unit 3 in the upper part of the sandstone complex (also called impact-tsunami). The finer grained layers contain multiple horizons abundantly bioturbated by Chondrites, Thalassinoides and Zoophycos (Fig. 17). |
Interpretation: The burrowed horizons indicate deposition over an extended time period during which invertebrates repeatedly colonized the ocean floor (Fig. 16). Detailed analysis of these burrowed horizons is given in Ekdale and Stinnesbeck (1998) and was first noted in Adatte et al. (1996), Stinnesbeck et al. (1996) and Keller et al. (l997). The presence of burrows is inconsistent with the tsunami hypothesis; no invertebrates could have been colonizing and happily burrow on the ocean floor during the few hours or days of the tsunami deposition. |
Argument in favor of impact-tsunami hypothesis: Ever since the NASA LPI sponsored fieldtrip in 1994 when Toni Ekdale, a world renown expert on trace fossils, discovered burrowed horizons in the so- called impact-tsunami deposit at El Mimbral and subsequently at El Penon and other localities Ekdale and Stinnesbeck, 1998), these burrows have been a sore issue with proponents of the impact-tsunami hypothesis. |
On that fieldtrip in 1994 Toni Ekdale’s discovery was publicly booed at the outcrop by Jan Smit, who loudly proclaiming to over 70 fieldtrip participants and over a dozen journalists, film and TV crews that no burrows exist and Toni had mistaken scratches with a hammer as trace fossils. Jan Smit is no trace fossil expert. Ever since that time Smit has denied the existence of burrows and trace fossils, even disingenuously claiming that Ekdale and Stinnesbeck (1998) had withdrawn their paper. They certainly did not. Their study stood the test as a careful and thorough analysis of trace fossils in the so-called impact-tsunami deposits of NE Mexico.
Unable to ignore the burrows in the El Penon sandstone complex, Smit argued that they are the result of downward burrowing from the KT boundary (e.g., Smit et al., l992, l996; Smit, l999).
Schulte et al. (2010, p. 1215) repeated Smit’s claims asserting that “A range of sedimentary structures and lack of evidence for ocean floor colonization within the clastic unit in northeastern Mexico indicate rapid deposition.” By denying the well documented evidence of trace fossils (e.g., Keller et al., 1997; Ekdale and Stinnesbeck, 1998) and asserting a range of undocumented sedimentary structures they reaffirmed the original 1992 conclusion of Smit et al. (1992) that the sandstone complex represents a tsunami deposit generated by the Chicxulub impact at the KT boundary.
It is hard to imagine that the irrational belief in the impact-tsunami hypothesis drives scientist to such desperate attempts to deny the obvious evidence – for no other reason than that it invalidates this hypothesis.
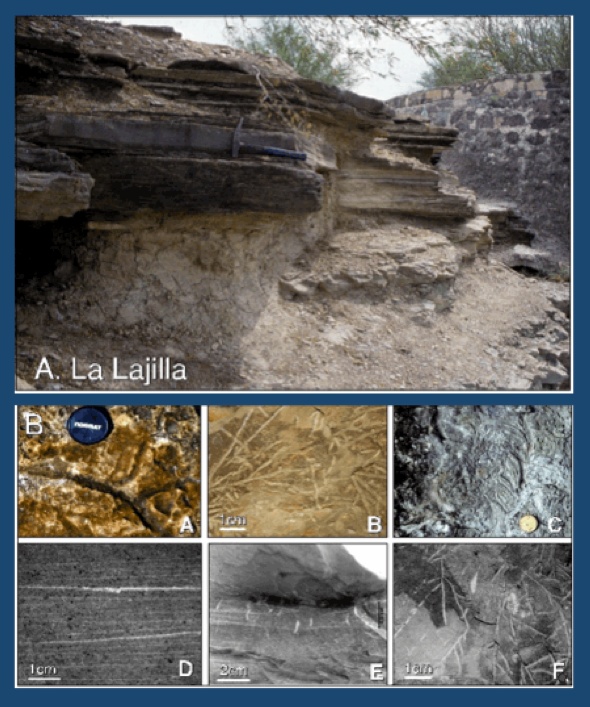
Figure 17. Alternating layers of silt and marly shale at the top part of the sandstone complex. The marly shale layers are laminated with mm thin lamina (D) and burrowed by Thalassinoides (A), Chondrites (B,E,F) and Zoophycos (C) From Keller, 2012.
Evidence: Marly shale layers of the alternating sand/shale deposits of unit 3 contain mm thin light and dark grey lamina (Fig. 17D). |
Interpretation: Such lamina are well-known as seasonal deposits accumulating just 1-2 millimeters each season. They thus represent deposition over many decades to hundreds and thousands of years of quiet seasonal deposition undisturbed by high-energy storms. In contrast, a tsunami is characterized by very high-energy wave action resulting in largely chaotic deposition and upward fining grain size, but never finely laminated dark and lighter grey seasonal deposits. This evidence also invalidates the impact-tsunami hypothesis. |
Argument in favor of impact-tsunami deposition: This sedimentary evidence is generally ignored by proponents of the impact-tsunami hypothesis. |
Evidence: At least two discrete zeolite-enriched layers are common in the alternating silt and shale layers (unit 3) as well as in the spherule-rich unit 1 at El Penon (Adatte et al., 1996, Fig. 18). |
Interpretation: These zeolite-enriched layers represent significant volcaniclastic influx. The fact that they are correlatable over long distances indicates discrete volcanic input during sedimentation of units 1 and 3. Such distinct layers are not compatible with high-energy tsunami deposition. The discrete volcanic input could not occur over the few hours to days of the tsunami event. The widespread presence of these zeolite-enriched layers indicate discrete times of volcanic input and times of normal sediment accumlation incompatible with a tsunami event. |
Argument in favor of impact-tsunami deposition: Proponents of the impact-tsunami hypothesis generally ignore this mineralogic evidence. |
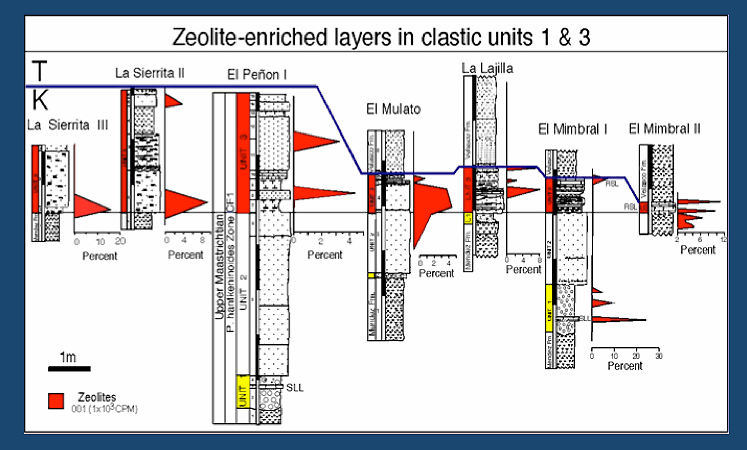
Figure 18. Correlation of the sandstone complex in NE Mexico showing the continuity of two zeolite-enriched layers across the region. These zeolite-enriched layers indicate volcanic input during depostion, which is inconsistent with tsunami deposition. From Adatte et al., 1996.
Sea – Level Change – Not Tsunami – Is The Answer
The well-documented trace-fossil horizons, zeolite layers, multiple spherule layers separated by limestone and other sedimentary features indicate sediment deposition over an extended time interval that cannot be explained by the impact-tsunami hypothesis. However, the latest Maastrichtian sea-level fall coincides with deposition of this sandstone complex and can easily explain the observed sedimentary features, including the scoured submarine channels. Already back in the early 1990s this alternative explanation was proposed by Keller, Adatte and Stinnesbeck based on the observed evidence (Stinnesbeck, 1993, 1996; Keller et al., 1994a; Keller and Adatte et al., 1996).
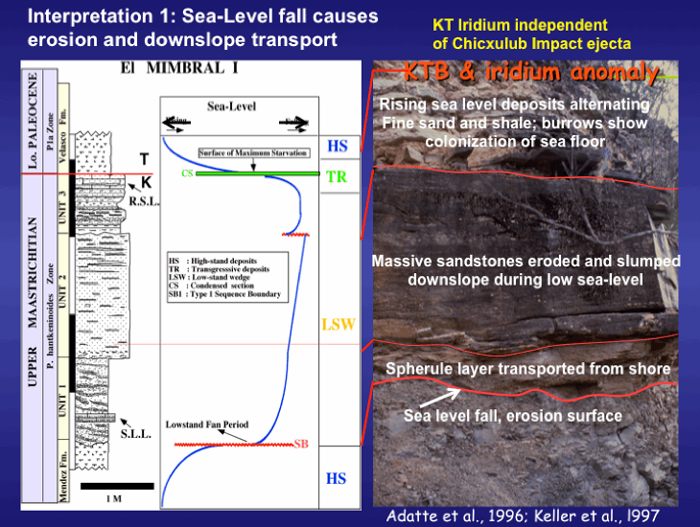
Figure 19. Sea-level fall can explain the sandstone complex in NE Mexico and account for the shallow water debris and glauconite in the spherule layer, the limestone that separates two spherule-rich layers, and the burrows and trace fossils. From Adatte et al., 1996; Keller et al., 1997.
Sea level changes are well documented for the KTB transition. In the case of the sandstone complex of NE Mexico (Fig. 19), the latest Maastrichtian sea level regression scoured submarine channels, eroded sediments in nearshore areas and transported these seaward across the shelf and downslope. The eroded and transported sediments included Chicxulub impact spherules deposited at some earlier time. The presence of two impact spherule layers separated by limestone in many localities indicates that there were repeated episodes of erosion and redeposition separated by normal (limestone) deposition. These deposits are labeled unit 1 in Fig. 19. Gravity slumps along the continental slope likely caused the influx of the massive sand unit 2. With the rising sea level, sand and shale layers of unit 3 were deposited. The sand layers mark periods of rapid sediment influx alternating with normal marly shale sedimentation during which invertebrates colonized the ocean floor (burrows in fine-grained layers; Fig. 17).
The iridium anomaly (Rocchia et al., 1996) and the KTB mass extinction (Keller et al., 1994b) at El Mimbral is in the clay layer above the sandstone complex and marks a condensed interval (surface of maximum starvation) followed by the continued rise in sea level (Adatte et al., 1996). The same lithological, faunal, and geochemical characteristics are observed in dozens of outcrops throughout northeastern Mexico (Keller et al., 2003a).